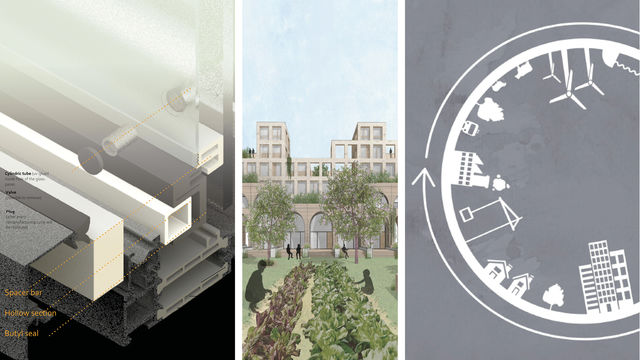
Rethinking Construction: The Circular Revolution
Could the buildings of the future be made from waste, plants, or even soil? At TU Delft, a new generation of architects is proving that sustainable construction isn’t just a dream—it's already taking shape.
The construction industry is one of the biggest contributors to CO₂ emissions and waste production. Yet, most building materials are rarely reused at scale. That’s why TU Delft challenges its students to rethink traditional construction and come up with bold, circular solutions. The Circularity in the Built Environment Graduation Awards highlight some of the most promising ideas. Here are five winning projects that could change the way we build.
1. Reviving Rammed Earth for Sustainable Housing
As cities expand and the demand for housing rises, the construction industry faces a growing dilemma: how to build efficiently without accelerating environmental damage. Concrete, the world’s most widely used construction material, is responsible for nearly 8% of global CO₂ emissions. Meanwhile, vast amounts of excavated soil are discarded as waste from construction and infrastructure projects. What if this «waste» could actually be the key to low-carbon, sustainable housing?
That’s the vision behind Larissa Götze's research at TU Delft. Her project, Let’s Build a Landfill, reimagines rammed earth—an ancient construction technique—as a modern, scalable solution for housing. By using excavated soil, she demonstrates how we can create durable, high-performance walls with minimal environmental impact.
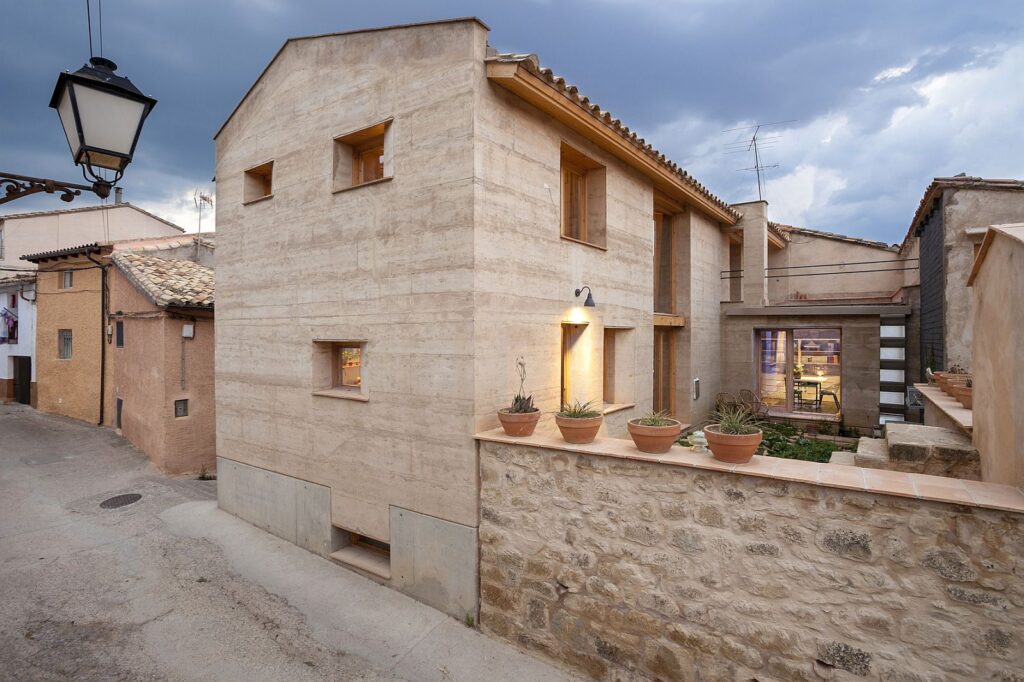
How Rammed Earth Works
Rammed earth construction involves compressing a precise mix of sand, silt, clay, and gravel into formwork, creating solid, load-bearing walls. When properly compacted, these walls become extremely dense and resilient, with high thermal mass that helps regulate indoor temperatures. Unlike concrete, rammed earth doesn’t require energy-intensive cement production, drastically cutting down on CO₂ emissions.
While traditional rammed earth structures are built on-site by manually tamping the material into layers, Larissa explored prefabrication as a way to streamline production. Her findings show that rammed earth panels can be factory-made, allowing for mass production and faster assembly on construction sites.
The Potential for Large-Scale Implementation
One of the biggest challenges to mainstream adoption of rammed earth is the perceived difficulty of scaling. It’s often seen as a niche, handcrafted technique rather than an industrial process. Larissa’s research challenges this assumption. By integrating robotic fabrication and modular design, she outlines a roadmap for turning existing concrete manufacturing facilities into rammed earth production hubs. This means:
- Lower costs through mass production and reduced labor intensity.
- Consistent quality with automated mixing and compaction techniques.
- Faster construction by delivering prefabricated panels directly to sites.
Instead of treating soil as waste, this approach transforms it into **a valuable, low-carbon building material** that can be used on a large scale.
Rammed Earth vs. Concrete: A Sustainable Alternative
Beyond its low carbon footprint, rammed earth offers several advantages over conventional materials:
- Durability — Ancient rammed earth structures, some over 1,000 years old, are still standing today.
- Energy Efficiency — The high thermal mass helps maintain stable indoor temperatures, reducing reliance on heating and cooling.
- Fire & Pest Resistance — Unlike wood, it is non-combustible and does not attract termites or mold.
- Local Material Use — Since soil is abundant in most regions, transportation emissions are significantly reduced.
A Vision for the Future
Larissa’s work proves that rammed earth is not just a historical technique—it's a viable, forward-thinking solution for today’s construction challenges. By adapting it to modern fabrication methods, it can become a mainstream alternative to carbon-heavy building materials.
With rising concerns about climate change, resource depletion, and housing shortages, redefining the way we build is no longer optional—it's essential. Rammed earth offers a compelling path forward, blending tradition with innovation to create sustainable, resilient homes for the future.
2. Local Crops as a Building Solution
As urban populations grow and the demand for housing increases, the construction industry faces pressure to build more—without further straining the environment. Traditional building materials like concrete and steel come with high carbon footprints, but what if the solution was growing all around us?
Frank Vahstal, a graduate of TU Delft, explored this question in his project 100,000 Biobased Top-Ups. His research focuses on biobased construction materials—building components made from locally sourced crops. By utilizing fast-growing plants like flax, hemp, and straw, he demonstrates how we can create sustainable housing solutions that reduce emissions and promote regional agriculture.
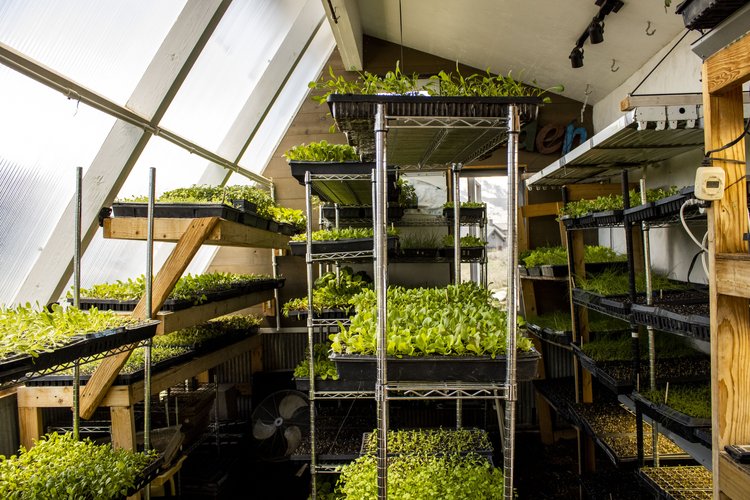
Why Use Crops for Construction?
Biobased materials offer multiple environmental advantages over conventional building materials:
- Carbon Storage — Plants absorb CO₂ during growth, effectively sequestering carbon within building materials.
- Renewability — Unlike finite resources like sand for concrete or iron ore for steel, crops can be regrown annually.
- Energy Efficiency — Many plant-based materials provide natural insulation, reducing heating and cooling needs.
- Waste Reduction — Agricultural byproducts, such as straw and seaweed, can be repurposed instead of discarded.
Shifting to locally sourced biobased materials could significantly cut emissions in the construction sector while supporting regional farmers and reducing dependency on carbon-heavy imports.
Turning Existing Buildings into Sustainable Spaces
One of the most sustainable approaches to urban expansion is topping up—adding extra floors to existing buildings instead of demolishing and rebuilding. This strategy maximizes land use without new concrete foundations, making it a low-impact way to create more living space.
Frank’s research takes this concept further by exploring biobased materials for these top-ups. He identified regionally suitable crops for different landscapes in the Netherlands:
- Clay soil — Ideal for flax and hemp cultivation.
- Sandy regions — Support the growth of seaweed and straw.
- Peatlands — Well-suited for cattail and pampas grass.
By sourcing materials directly from the land, this approach creates a closed-loop system, where construction supports agriculture and vice versa.
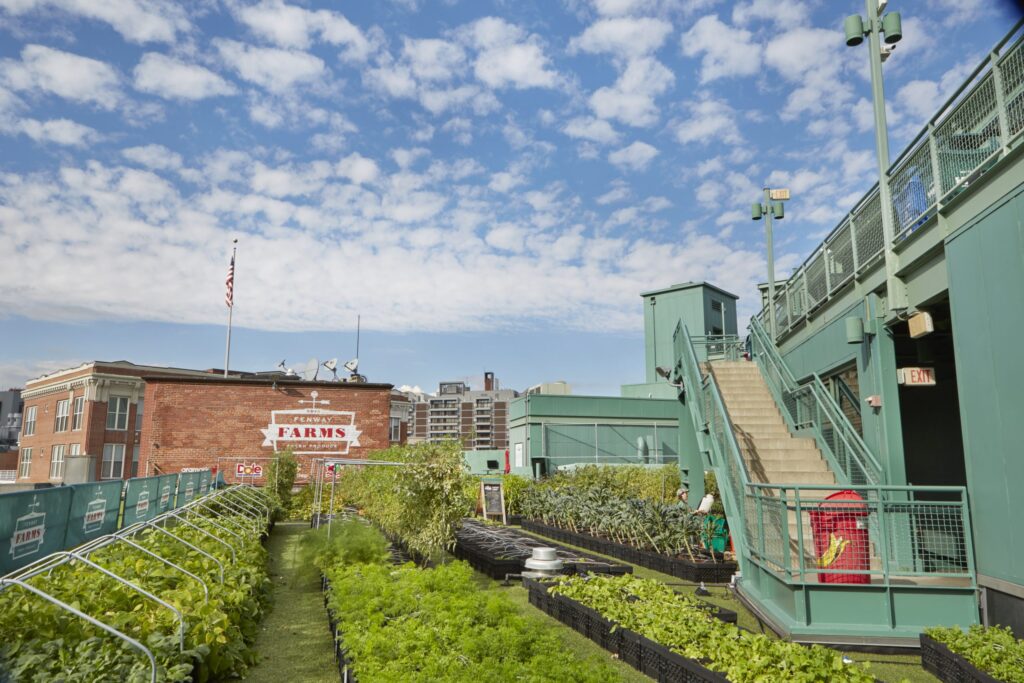
Scaling Up: The Challenge of Biobased Construction
While biobased materials have clear environmental benefits, they still face barriers to mass adoption:
- Processing & Standardization — Unlike steel and concrete, plant-based materials vary in texture and durability, requiring specialized treatment.
- Market Readiness — The construction industry is slow to change, and regulatory frameworks still favor traditional materials.
- Perceived Durability — Many builders remain skeptical about the long-term performance of plant-based components.
Despite these challenges, Frank’s research offers a framework for integrating biobased construction into mainstream housing development. With the right policies and investment in processing infrastructure, these materials could become a key player in sustainable urban expansion.
A Future Built with Nature
The construction industry is at a crossroads—continue relying on high-emission materials or embrace circular, nature-based solutions. Frank’s work highlights that biobased top-ups are not just an ecological alternative but a practical, scalable solution to housing shortages.
With local crops as building materials, cities can grow sustainably, reducing their carbon footprint while fostering regional economies. The future of construction doesn’t just lie in factories—it might just be in the fields.
3. A Circular System for Neodymium Magnets
Neodymium magnets are everywhere—from wind turbines and electric vehicles to smartphones and medical devices. They are essential for modern technology, yet their production relies on rare earth mining, a process that is not only expensive but also environmentally destructive. With demand for these magnets surging, how can we make their supply more sustainable?
That’s the question Preksha Rautela explored in her project Extractivism to Circularism. She investigated how the Netherlands could establish a closed-loop system for neodymium magnets, reducing reliance on raw material extraction and minimizing environmental harm.
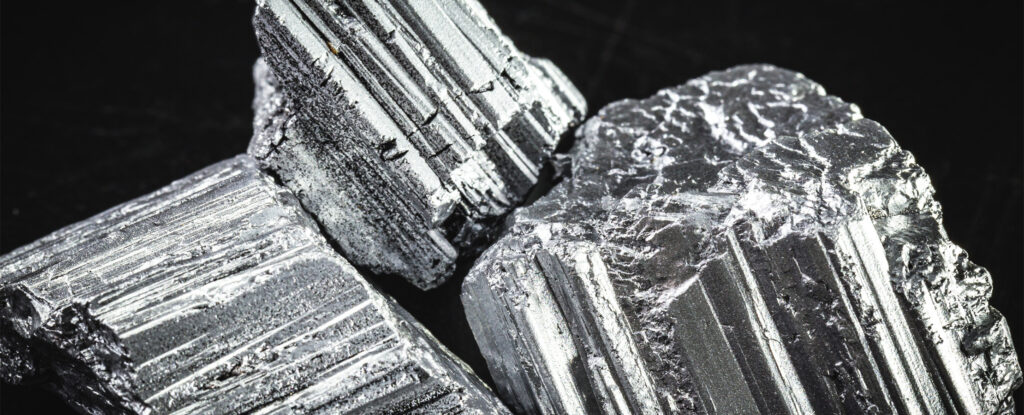
The Problem with Neodymium Mining
Neodymium is a rare earth element primarily mined in China, the U.S., and Australia. The extraction process is energy-intensive, generates toxic waste, and often leads to water and soil contamination. Additionally, because these magnets are embedded in complex electronics, recycling them is difficult, and most end up in landfills. <blockquote>Without a circular system in place, discarded neodymium magnets represent a **massive loss of valuable material**—one that could otherwise be reused for new technology.</blockquote>
A Circular Supply Chain for Neodymium
Preksha proposed four different scenarios for creating a sustainable neodymium cycle in the Netherlands. Each scenario considered:
- Collection & Recovery — Setting up specialized e-waste recycling hubs to extract magnets from old electronics.
- Processing & Refining — Developing localized facilities to purify and recondition used neodymium.
- Manufacturing & Reuse — Implementing a system where recovered magnets are remanufactured into new products.
- Infrastructure & Feasibility — Assessing the land, energy, and environmental impact of a circular supply chain.
Her research identified key locations where recycling facilities could be established, ensuring efficient logistics and minimal transportation emissions.
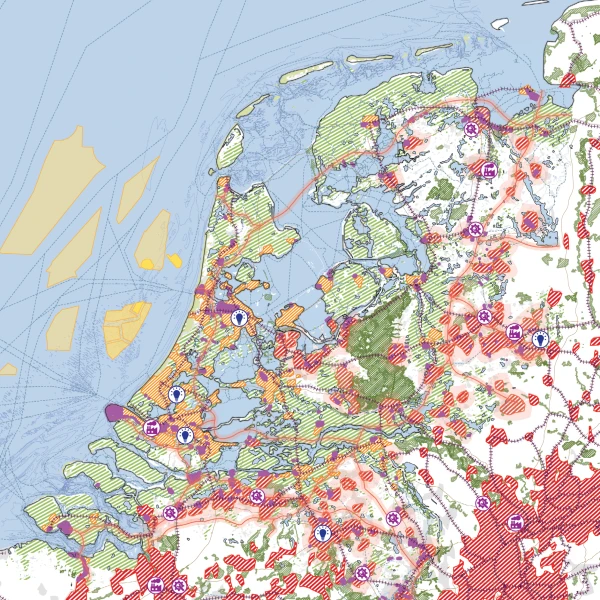
The Potential Impact of Circular Neodymium
If implemented, a circular system for neodymium magnets could:
- Reduce reliance on rare earth mining, lowering environmental damage.
- Decrease e-waste, keeping valuable materials in circulation.
- Enhance supply chain security, making Europe less dependent on imports.
- Cut production costs by reusing existing materials rather than extracting new ones.
Beyond Neodymium: A Blueprint for Other Critical Materials
One of the most valuable aspects of Preksha’s research isn’t just the outcome—it's the scenario-building method she developed. This approach can be applied to other critical raw materials, such as lithium (used in batteries) or indium (found in touchscreens), helping create more circular supply chains across industries.
As demand for rare earth elements continues to grow, circular strategies like Preksha’s will be essential for reducing environmental impact and ensuring long-term sustainability. The future of technology doesn’t have to rely on endless extraction—it can be built on smarter, closed-loop solutions.
4. Circular Neighborhoods for a Greener Future
Many post-war neighborhoods were built quickly to address housing shortages, often prioritizing efficiency over sustainability. Decades later, these areas face aging infrastructure, inefficient energy use, and limited green spaces. However, instead of demolishing and rebuilding, what if these neighborhoods could be transformed into self-sustaining circular communities?
This is precisely what Elena Grimbacher explored in her project Circularity of the Everyday. Focusing on the post-war Schalkwijk neighborhood in Haarlem, she developed a pattern language—a design framework that allows urban planners, policymakers, and local residents to integrate circular systems for water, energy, and food production.
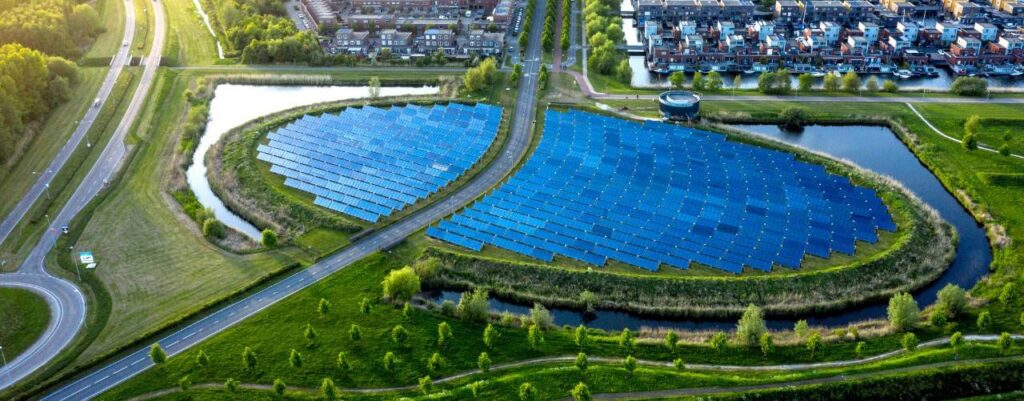
The Challenge of Post-War Neighborhoods
Built in the 1950s and 1960s, these neighborhoods often share common characteristics:
- Outdated infrastructure that leads to energy inefficiencies.
- Minimal biodiversity, with large, underutilized green spaces.
- Disconnected waste and water management systems.
- Reliance on centralized energy sources, increasing emissions.
<blockquote>Instead of treating these issues separately, a circular neighborhood integrates **water, energy, food, and waste systems**, creating a self-sustaining urban environment.</blockquote>
Rethinking Green Spaces: From Lawns to Productive Landscapes
Many post-war neighborhoods feature expansive, unused lawns between apartment buildings. While they offer greenery, they often lack biodiversity and practical function. Elena’s project envisions transforming these spaces into:
- Community vegetable gardens that provide fresh produce and strengthen local food systems.
- Orchards with fruit trees, offering natural shade and seasonal harvests.
- Urban wetlands and rain gardens, which help absorb excess rainwater, preventing flooding.
This shift enhances biodiversity, improves local food security, and reduces heat island effects, making neighborhoods more resilient to climate change.

Integrating Circular Water & Energy Systems
Elena’s approach extends beyond landscaping—she explores how neighborhoods can become self-sufficient in water and energy use. Her vision includes:
- Rainwater harvesting for irrigation and household use.
- Greywater recycling systems, filtering water for reuse in homes and gardens.
- Solar panels & district heating, reducing dependency on fossil fuels.
These systems, when combined, reduce strain on municipal resources and lower environmental impact.
A Scalable Model for Other Cities
Elena’s research isn’t just about Schalkwijk—it's a replicable model for other post-war neighborhoods across Europe. By using her pattern language, urban planners can:
- Adapt circular strategies to local conditions.
- Engage communities in the design process.
- Create more livable, self-sustaining urban environments.
The future of urban development doesn’t have to mean starting from scratch. By retrofitting existing neighborhoods with circular principles, cities can preserve their architectural heritage while creating greener, more resilient communities.
5. Facade Panels Made from Walnut Shells
What if food waste could be repurposed into high-performance building materials? That’s exactly what Lara Neuhaus set out to explore in her project Bio-Composites from Food Waste. Her research focused on developing a durable, rainproof facade panel made from walnut shells—a byproduct that is usually discarded.
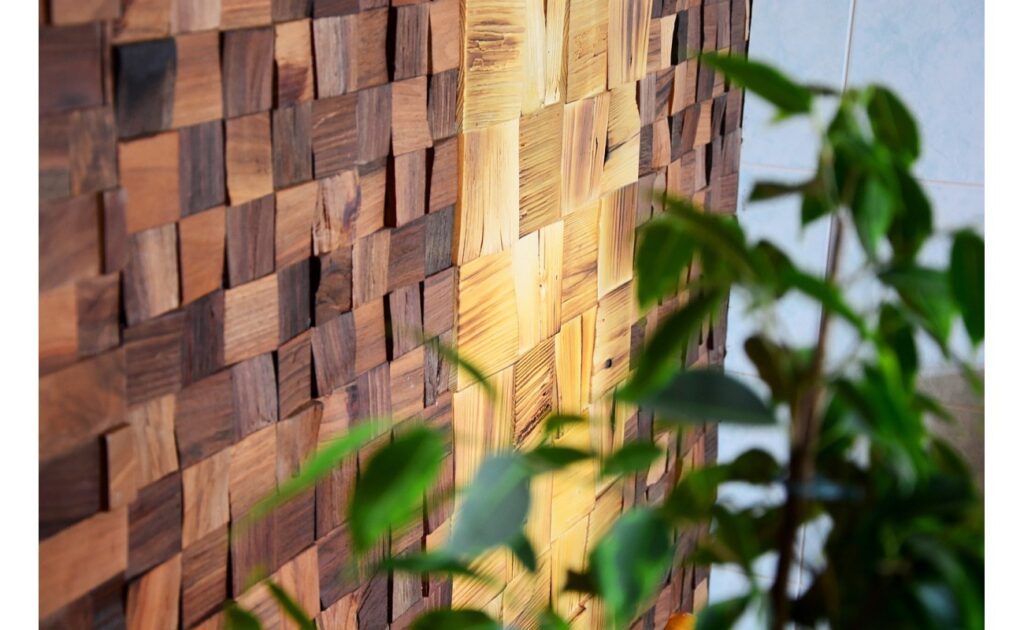
Turning Waste into Building Materials
The food industry generates millions of tons of organic waste each year. While some of it is composted, much of it still ends up in landfills or incinerators, contributing to carbon emissions. Lara’s project takes a different approach: using walnut shells—which are abundant, strong, and naturally weather-resistant—as the base for a biodegradable composite.
By transforming food waste into **sustainable building materials**, we reduce landfill waste while creating alternatives to carbon-intensive materials like concrete and plastic.
How Walnut Shell Panels Are Made
To create her bio-composite, Lara tested various food waste streams, including coffee grounds and nut shells. Through extensive material testing, she found that walnut shells, when combined with a biobased resin and compressed under high heat and pressure, produced a strong, weather-resistant material. The process involves:
- Collecting & Processing — Walnut shells are cleaned, crushed, and mixed with a biodegradable binding agent.
- Compression & Heating — The mixture is pressed into panels at 150°C, creating a solid, durable surface.
- Testing & Optimization — The panels are evaluated for strength, water resistance, and thermal insulation properties.
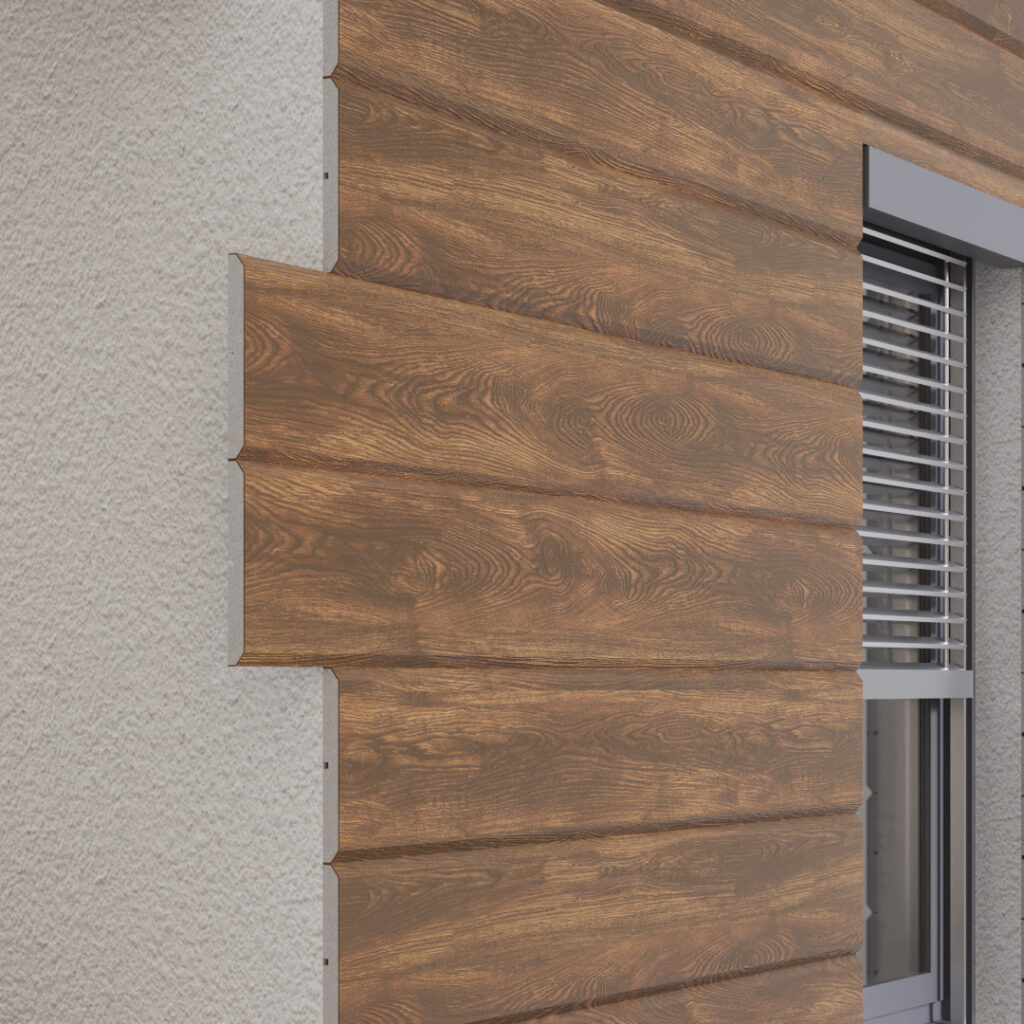
Why Walnut Shells?
Among the materials tested, walnut shell composite showed the best performance in terms of:
- Water & frost resistance — Making it suitable for exterior facades.
- Durability & impact resistance — Comparable to some traditional building materials.
- Carbon footprint — Unlike wood, which needs years to grow, walnut shells are an agricultural byproduct, making them an immediately available, renewable resource.
A Sustainable Alternative to Conventional Facades
Traditional facade materials, such as cement-based panels or aluminum cladding, require high-energy production processes and contribute to CO₂ emissions. Walnut shell panels, on the other hand, offer:
- A natural, biodegradable alternative that doesn’t rely on virgin resources.
- Circular potential, as old panels can be broken down and reused.
- Aesthetic appeal, with a unique organic texture that brings a natural look to buildings.
The Future of Bio-Based Construction
Lara’s research is part of a growing movement toward bio-based building materials, proving that waste doesn’t have to be waste. By scaling up production, walnut shell panels could become a mainstream, eco-friendly alternative for architects and builders looking to reduce their environmental impact.
As the construction industry shifts toward circularity, materials like walnut shell composites could play a key role in redefining sustainability in architecture—one building at a time.
A Future Built on Circularity
The construction industry is at a turning point. As these five projects demonstrate, sustainable solutions exist—it's just a matter of scaling them up. From rethinking traditional materials to designing entire circular neighborhoods, TU Delft students are showing the world what’s possible.
If these innovations gain traction, the future of construction won’t just be greener—it will be circular.